Demantia
Dementia is a brain condition. It affects memory, thinking, and behavior. It makes daily tasks difficult. It usually occurs in older people, but not restricted to older people. There is no complete cure as yet, but treatment can help manage symptoms.
Dementia is NOT a term indicating any single symptom and single root cause. It is a kind of an umbrella term that describes a wide varieties of symptoms associated with wide range of root causes.
There are wide varieties of symptoms associated with demantia. What kind of symptoms would appear would vary depending on persons, but we can list up symptoms that are most commonly observed.
- Memory issues: People might forget things often, like where they put their keys or what they did yesterday.
- Confusion: They could get mixed up with time, dates, or even familiar places.
- Problem-solving: Simple tasks like paying bills or following a recipe can become difficult.
- Speaking: They may struggle to find words or join conversations.
- Mood changes: It's common to see mood swings, like getting sad or angry easily.
- Trouble concentrating: People with dementia may have a hard time focusing on tasks or following a train of thought.
- Misplacing items: They might put things in unusual places, like a wallet in the fridge or glasses in a drawer.
- Social withdrawal: They may lose interest in social activities or hobbies they used to enjoy.
- Poor judgment: Dementia can affect decision-making, leading to mistakes or risky choices.
- Personality changes: People may become more irritable, suspicious, or fearful than before.
Following images shows an example of Alzheimer patient's brain scan representing GCA. GCA stands for Global Cortical Atrophy. The GCA scale is a semi-quantitative rating system used to assess the degree of cortical atrophy visible on brain scans of patients with dementia. Cortical atrophy refers to the shrinking or loss of brain tissue in the cerebral cortex, the outer layer of the brain responsible for many higher cognitive functions. This atrophy can be indicative of neurodegenerative diseases
such as Alzheimer's disease, frontotemporal dementia, and other types of dementia.
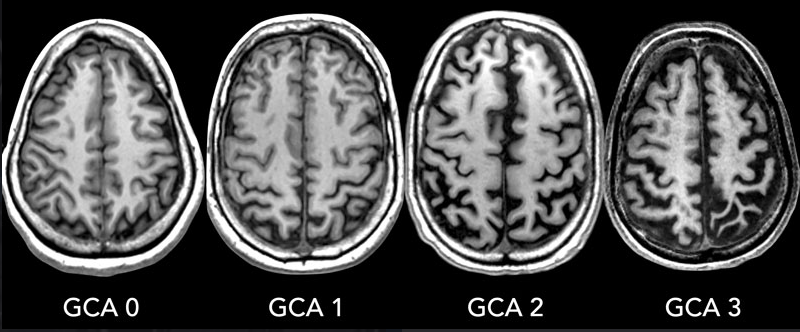
Image source : Dementia - Role of MRI
The GCA scale typically ranges from 0 to 3, with higher scores representing greater atrophy:
- GCA 0: No significant cortical atrophy.
- GCA 1: Mild cortical atrophy. There is a slight widening of the sulci (the grooves on the surface of the brain) and a mild reduction in the volume of the gyri (the ridges on the surface of the brain).
- GCA 2: Moderate cortical atrophy. There is a more pronounced widening of the sulci and a reduction in the volume of the gyri. The ventricles, the fluid-filled spaces within the brain, may also appear enlarged.
- GCA 3: Severe cortical atrophy. The sulci are significantly widened, and the gyri are substantially reduced in volume. The ventricles may appear severely enlarged.
NOTE : Cognitive/behavioral symptoms of GCA level
The Global Cortical Atrophy (GCA) scale is used to assess the severity of cortical atrophy, which is common in various forms of dementia. Here is a list of common cognitive and behavioral symptoms associated with each stage of GCA:
- GCA Stage 0 (No Cortical Atrophy)
- Cognitive Symptoms: Normal cognitive function; no signs of memory loss or cognitive impairment.
- Behavioral Symptoms: None, as the individual functions normally without any noticeable changes.
- GCA Stage 1 (Mild Cortical Atrophy)
- Cognitive Symptoms: Very mild cognitive decline, such as occasional lapses in memory (e.g., forgetting where everyday objects are placed, or familiar names). These changes are often too subtle to interfere with daily life or be detected in a clinical interview.
- Behavioral Symptoms: Increased frequency of minor forgetfulness, which might cause slight frustration or anxiety but typically does not impact work or social interactions.
- GCA Stage 2 (Moderate Cortical Atrophy)
- Cognitive Symptoms: Mild cognitive decline becomes more noticeable. Symptoms include getting lost in familiar places, difficulty retaining new information, forgetting names of people just met, misplacing valuable items, trouble concentrating, and decreased performance at work.
- Behavioral Symptoms: Increased anxiety, difficulty in social settings, and growing awareness of cognitive difficulties. Individuals might start to avoid challenging situations to hide their cognitive issues.
- GCA Stage 3 (Severe Cortical Atrophy)
- Cognitive Symptoms: Moderate cognitive decline. Individuals show significant deficits in memory, such as forgetting personal history details, and have difficulty organizing and planning daily activities. They might be disoriented about time and place.
- Behavioral Symptoms: Higher levels of anxiety, frustration, and depression due to the noticeable impact on daily functioning. There might be personality changes, such as increased irritability or withdrawal from social interactions.
Following is a pair of MRI image comparing crosecion of the brain for a normal person and a dementia(Alzheimer) patient. As you would notice, brain is shrinked overall (showing more of black area) and himpocampus (highlighed in red) are damaged in the patient's brain.
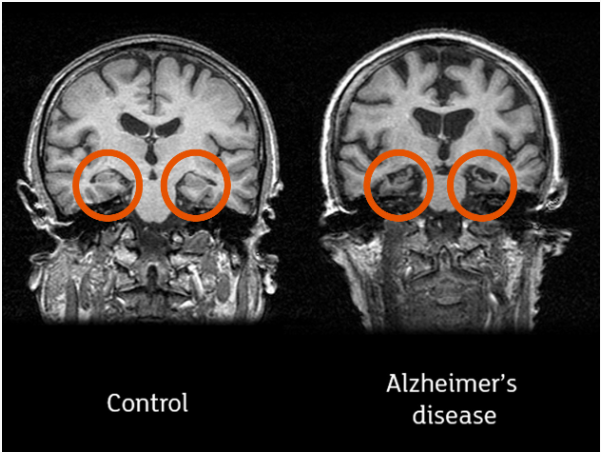
Image Source : All you need to know about brain scans and dementia
Following is a pair of PET image comparing brain activity for a normal person and a dementia(Alzheimer) patient. As you would notice, the patient's brain shows less active (showing less area of bright colors) and more Amyloid accumulation (more area of bright colors).
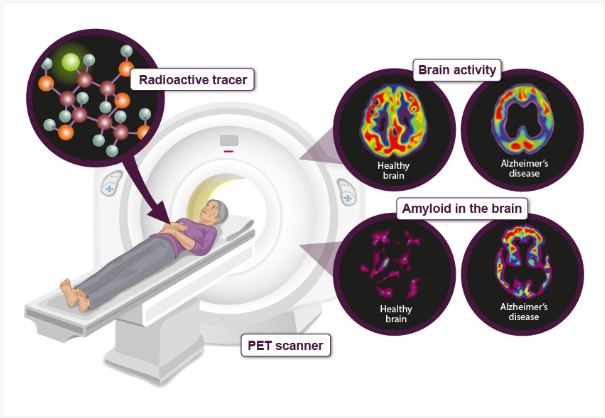
Image Source : All you need to know about brain scans and dementia
Dementia is not a single disease but a general term for a decline in mental ability severe enough to interfere with daily life. It is caused by damage to or loss of nerve cells and their connections in the brain. There are many different causes of dementia, each with its own set of symptoms and progression.
Common Causes of Dementia:
The most prevalent causes of dementia involve various neurodegenerative diseases and vascular conditions that progressively damage brain cells. These conditions are responsible for the majority
of dementia cases and typically lead to a gradual decline in cognitive function and memory.
Other Causes of Dementia:
Apart from the more common types, there are several other causes of dementia that may be less frequent but still significantly impact cognitive health. These include genetic disorders, mixed forms of dementia, and other neurodegenerative diseases that can manifest in unique and complex ways.
-
Parkinson’s Disease
-
Description: A neurodegenerative disorder primarily affecting movement.
-
Pathology: Parkinson’s disease dementia occurs as the disease progresses, affecting cognitive functions.
-
Symptoms: Similar to Lewy body dementia, including visual hallucinations, impaired memory, and motor dysfunctions.
-
Huntington’s Disease
-
Description: A genetic disorder that causes the progressive breakdown of nerve cells in the brain.
-
Pathology: Huntington's disease is caused by a genetic mutation that leads to widespread brain degeneration.
-
Symptoms: Cognitive decline, personality changes, and uncontrolled movements (chorea).
-
Mixed Dementia
-
Description: This occurs when a person has more than one type of dementia simultaneously, such as Alzheimer’s disease and vascular dementia.
-
Pathology: The coexistence of multiple types of brain abnormalities.
-
Symptoms: A combination of symptoms from the different types of dementia present.
-
Other Less Common Causes
-
Creutzfeldt-Jakob Disease: A rare, degenerative brain disorder caused by prions, leading to rapid mental deterioration.
-
Normal Pressure Hydrocephalus: An accumulation of cerebrospinal fluid in the brain's ventricles, causing symptoms similar to dementia.
-
Infections: Certain infections such as HIV and syphilis can lead to cognitive impairment if untreated.
Reversible Causes of Dementia-like Symptoms:
Not all conditions that mimic dementia are permanent. Some can be reversed with appropriate treatment, leading to a restoration of normal cognitive function. These reversible causes often stem from metabolic imbalances, vitamin deficiencies, infections, and other treatable medical conditions.
The biochemical mechanism of amyloid plaque formation involves several steps and key molecules, primarily revolving around the processing of amyloid precursor protein (APP).
Amyloid plaque formation is a complex, multistep process involving the abnormal processing of APP, leading to the production and aggregation of Aβ peptides. These peptides aggregate to form oligomers and fibrils, ultimately resulting in extracellular amyloid plaques associated with neurotoxicity and Alzheimer's disease pathology.
1. Amyloid Precursor Protein (APP) Processing:
-
APP Structure: APP is a transmembrane protein expressed in many tissues, especially in the brain.
-
Proteolytic Processing: APP can be cleaved by different enzymes through two main pathways: the non-amyloidogenic pathway and the amyloidogenic pathway.
2. Non-Amyloidogenic Pathway:
-
Alpha-Secretase Cleavage: In this pathway, alpha-secretase cleaves APP within the amyloid-beta (Aβ) region, preventing Aβ formation. This results in the production of soluble APP alpha (sAPPα) and a membrane-bound fragment called C83.
-
Gamma-Secretase Cleavage: Gamma-secretase further cleaves C83, producing a small peptide called p3 and the APP intracellular domain (AICD), both of which are not amyloidogenic.
3. Amyloidogenic Pathway:
-
Beta-Secretase Cleavage (BACE1): In this pathway, beta-secretase cleaves APP at the N-terminus of the Aβ region, producing soluble APP beta (sAPPβ) and a membrane-bound fragment called C99.
-
Gamma-Secretase Cleavage: Gamma-secretase cleaves C99 within the membrane, producing Aβ peptides of varying lengths (most commonly Aβ40 and Aβ42) and the APP intracellular domain (AICD).
4. Aggregation of Aβ Peptides:
-
Monomer Formation: Initially, Aβ peptides exist as monomers.
-
Oligomerization: Aβ monomers can aggregate to form soluble oligomers, which are believed to be toxic and are implicated in synaptic dysfunction.
-
Fibrillogenesis: Soluble oligomers can further aggregate to form insoluble fibrils, which eventually deposit extracellularly as amyloid plaques.
5. Plaque Formation:
-
Plaque Core: Amyloid plaques typically have a dense core composed of fibrillar Aβ.
-
Surrounding Tissue: Plaques are surrounded by dystrophic neurites, activated microglia, and reactive astrocytes, indicating a local inflammatory response.
-
Plaque Maturation: Over time, plaques can become more complex and include additional components such as metals (e.g., zinc, copper), glycosaminoglycans, and other proteins.
6. Neurotoxic Effects:
-
Synaptic Dysfunction: Soluble Aβ oligomers interfere with synaptic function, leading to impaired neurotransmission and cognitive deficits.
-
Neuroinflammation: Microglial activation and astrocytic response contribute to chronic inflammation, exacerbating neuronal damage.
-
Cell Death: Persistent toxicity and inflammation can lead to neuronal loss and the characteristic brain atrophy seen in Alzheimer's disease.
NOTE : What is normal functionality of Amyloid protein before it turned into amyloid beta ?
-
The amyloid precursor protein (APP) has several normal physiological functions before it is cleaved to form amyloid-beta (Aβ).
-
Neuronal Growth and Repair: APP plays a role in neuronal development, growth, and repair processes, aiding in the maintenance and regeneration of neural tissues.
-
Synaptic Function: APP is involved in synaptic formation and plasticity, which are critical for learning and memory.
-
Cell Adhesion: APP contributes to cell adhesion, helping cells stick together and communicate effectively.
-
Neuroprotection: APP may have protective effects on neurons, helping to shield them from various forms of cellular stress and injury.
-
Intracellular Signaling: APP is involved in intracellular signaling pathways that regulate various cellular functions, including gene expression and cell survival.
While the exact mechanisms of APP function are still under investigation, it is clear that this protein plays a crucial role in neuronal development, function, and survival. However, when APP is cleaved by specific enzymes, it can generate Aβ fragments, which are prone to aggregation and can lead to the formation of amyloid plaques in Alzheimer's disease. This pathological process disrupts the normal functions of APP and contributes to neurodegeneration.
The biochemical mechanism of tau protein formation involves its synthesis, post-translational modifications, and aggregation, particularly in the context of tauopathies like Alzheimer's disease. Tau protein formation and its pathological aggregation involve a complex interplay of gene expression, post-translational modifications, particularly hyperphosphorylation, and subsequent aggregation into toxic oligomers and neurofibrillary tangles. These processes disrupt neuronal function
and contribute to the neurodegenerative changes observed in diseases like Alzheimer's.
1. Tau Protein Synthesis:
-
Gene Encoding: Tau protein is encoded by the MAPT (Microtubule-Associated Protein Tau) gene located on chromosome 17.
-
Alternative Splicing: The MAPT gene undergoes alternative splicing, producing six different isoforms of tau in the adult human brain. These isoforms vary in the number of microtubule-binding domains and N-terminal inserts.
2. Tau Protein Function:
-
Microtubule Stabilization: Tau proteins primarily function to stabilize microtubules, which are essential for maintaining the structure and function of neurons. They achieve this by binding to tubulin in microtubules.
-
Axonal Transport: Tau proteins facilitate axonal transport by supporting the assembly and stability of microtubules, crucial for transporting cellular cargo along axons.
3. Post-Translational Modifications:
-
Phosphorylation: The most critical modification of tau is phosphorylation. Under normal conditions, tau is phosphorylated at several sites, which regulates its binding to microtubules. In pathological conditions, hyperphosphorylation occurs.
-
Other Modifications: Tau can also undergo acetylation, ubiquitination, glycation, and truncation, among other modifications, which can affect its function and aggregation propensity.
4. Hyperphosphorylation:
-
Kinases Involved: Hyperphosphorylation of tau is mediated by various kinases, such as GSK-3β (glycogen synthase kinase 3 beta), CDK5 (cyclin-dependent kinase 5), and others.
-
Consequences: Hyperphosphorylated tau has a reduced affinity for microtubules, leading to their destabilization. This results in the disassembly of the microtubule network and impaired axonal transport.
5. Tau Aggregation:
-
Monomeric Tau: Normally, tau exists in a soluble, monomeric form.
-
Oligomerization: Under pathological conditions, hyperphosphorylated tau aggregates into soluble oligomers. These oligomers are thought to be toxic and interfere with neuronal function.
-
Fibril Formation: Oligomers can further aggregate into paired helical filaments (PHFs) and straight filaments, which are the building blocks of neurofibrillary tangles (NFTs).
6. Neurofibrillary Tangle (NFT) Formation:
-
Tangle Structure: NFTs are intracellular aggregates of tau filaments found in the neuronal cell bodies. These tangles disrupt normal cellular function and contribute to neuronal death.
-
Tangle Maturation: Over time, NFTs can mature and become more insoluble, leading to severe disruptions in cellular architecture and function.
7. Neurotoxic Effects:
-
Synaptic Dysfunction: Tau oligomers and NFTs impair synaptic function, contributing to cognitive deficits and memory loss.
-
Cellular Stress: Accumulation of abnormal tau induces cellular stress responses, including mitochondrial dysfunction, oxidative stress, and activation of apoptotic pathways.
-
Neuronal Death: Persistent tau pathology leads to neuronal degeneration and brain atrophy, which are characteristic of tauopathies like Alzheimer's disease and frontotemporal dementia.
NOTE : What is the normal functionality of normal tau protein ?
Tau protein, in its normal state, plays a crucial role in maintaining the structure and function of neurons, particularly in the axons. Its primary functions include:
-
Microtubule Stabilization: Tau's most well-known function is to bind to and stabilize microtubules, which are long, cylindrical structures that form the internal skeleton of neurons. These microtubules act as tracks for transporting essential molecules and organelles along the axon, ensuring proper neuronal communication.
-
Regulation of Microtubule Dynamics: Tau not only stabilizes microtubules but also regulates their assembly and disassembly. This dynamic regulation is crucial for neuronal growth, plasticity, and response to changes in the environment.
-
Axonal Transport: By stabilizing microtubules, tau indirectly facilitates the efficient transport of various cargoes, including neurotransmitters, organelles, and growth factors, along the axon. This transport is essential for maintaining neuronal function and survival.
-
Signaling and Scaffolding: Tau can also interact with other proteins involved in signaling pathways and cellular processes. It can act as a scaffold, bringing together different proteins and molecules to facilitate their interactions and regulate their functions.
-
Neurite Outgrowth and Synaptic Function: Tau has been implicated in neurite outgrowth, the process by which neurons extend their axons and dendrites. It may also play a role in synaptic function, influencing the communication between neurons.
Overall, tau protein is a multifunctional protein that plays a vital role in maintaining the health and function of neurons. Its dysfunction and aggregation, as seen in neurodegenerative diseases like Alzheimer's, disrupt these essential functions and contribute to neuronal damage and cognitive decline.
There is growing evidence that amyloid-beta (Aβ) plays a significant role in the abnormal tau protein formation observed in neurodegenerative diseases like Alzheimer's.
Overall, Aβ appears to act as an upstream trigger for abnormal tau formation, but tau can also exacerbate Aβ-induced damage. This creates a vicious cycle of Aβ and tau interaction, contributing to the progressive neurodegeneration seen in Alzheimer's.
Amyloid-induced Tau Hyperphosphorylation:
-
Aβ oligomers can activate enzymes called kinases, which are responsible for adding phosphate groups to tau protein. This excessive phosphorylation, known as hyperphosphorylation, disrupts tau's normal function and promotes its aggregation.
-
Aβ can also inhibit phosphatases, the enzymes that remove phosphate groups, further exacerbating tau hyperphosphorylation.
Amyloid-mediated Tau Misfolding and Aggregation:
-
Aβ oligomers can directly interact with tau protein, altering its shape and promoting its misfolding. This misfolded tau is prone to clumping together, forming neurofibrillary tangles (NFTs) - a hallmark of Alzheimer's.
-
Aβ can also disrupt cellular processes that normally prevent tau misfolding, like the proteasome system responsible for breaking down abnormal proteins.
Amyloid-triggered Neuroinflammation:
-
Aβ deposits in the brain can trigger an immune response, activating microglia and astrocytes. These cells release inflammatory molecules that further promote tau hyperphosphorylation and aggregation.
-
Inflammation can also lead to oxidative stress, which can damage tau and make it more likely to misfold.
Amyloid-induced Synaptic Dysfunction:
Evidence from Studies:
-
Animal models of Alzheimer's, where Aβ is overproduced, often show increased tau pathology, suggesting a direct link.
-
Human studies using brain imaging and biomarkers have found correlations between Aβ burden and tau pathology in Alzheimer's patients.
-
Some clinical trials targeting Aβ have shown modest effects on tau pathology in early-stage Alzheimer's.
Followings are the list of medications listed by Dementia (World Health Organization)
- Cholinesterase inhibitors like donepezil are used to treat Alzheimer disease.
- NMDA receptor antagonists like memantine are used for severe Alzheimer disease and vascular dementia.
- Medicines to control blood pressure and cholesterol can prevent additional damage to the brain due to vascular dementia.
- Selective serotonin reuptake inhibitors (SSRIs) can help with severe symptoms of depression in people living with dementia if lifestyle and social changes dont work, but these should not be the first option.
Followings are the list of medication approved by FDA. The list came from Medications for Memory, Cognition and Dementia-Related Behaviors
- Drugs that change disease progression
- Aducanumab : monoclonal antibody that targets amyloid-beta plaques
- Lecanemab : monoclonal antibody that targets amyloid-beta plaques
- Drugs that treat symptoms
- Cholinesterase inhibitors (Donepezil, Rivastigmine, Galantamine) : increasing the levels of acetylcholine, a neurotransmitter involved in memory and learning, in the brain
- Glutamate regulators (Memantine) : regulating the activity of glutamate, a neurotransmitter involved in learning and memory.
- Cholinesterase inhibitor + glutamate regulator (Donepezil and memantine) : enhancing the effects of acetylcholine while also regulating glutamate levels, potentially resulting in improved cognitive function and slower disease progression
- Orexin receptor antagonist (Suvorexant) : treating sleep disorders, like insomnia
NOTE : These medications are usually used to mitigate the symptoms. They do not cure dementia.
Biochemical/physiological mechanism of Cholinesterase inhibitors
Cholinesterase inhibitors (ChEIs) are a class of drugs used to treat dementia, particularly Alzheimer's disease. They work by targeting the cholinergic system in the brain, which is significantly affected in dementia. Here's how they work:
Biochemical Mechanism:
-
Acetylcholine (ACh) and Cholinesterase: ACh is a neurotransmitter crucial for memory, learning, and other cognitive functions. It's released by neurons and binds to receptors on other neurons to transmit signals. Cholinesterase is an enzyme that breaks down ACh in the synaptic cleft (the space between neurons), terminating its action.
-
Inhibition of Cholinesterase: ChEIs inhibit the activity of cholinesterase. This prevents the breakdown of ACh, leading to increased levels of ACh in the synaptic cleft.
-
Enhanced Cholinergic Transmission: The higher concentration of ACh allows it to bind to more receptors, enhancing cholinergic transmission. This can improve cognitive function, albeit temporarily, in patients with dementia.
Physiological Mechanism:
-
Neuronal Communication: By increasing ACh levels, ChEIs enhance communication between neurons in brain regions involved in memory and cognition. This can help compensate for the loss of neurons and synapses that occur in dementia.
-
Neuroprotection: ChEIs may also have neuroprotective effects by reducing the production of amyloid-beta (Aβ) peptides, which are involved in the formation of amyloid plaques, a hallmark of Alzheimer's disease.
-
Anti-inflammatory Effects: Some ChEIs have anti-inflammatory properties, which may help to protect neurons from damage caused by inflammation.
Limitations:
-
Symptomatic Treatment: ChEIs do not cure dementia or reverse the underlying neurodegenerative process. They primarily offer symptomatic relief and may slow down cognitive decline.
-
Limited Efficacy: The effectiveness of ChEIs varies among individuals and depends on the stage of dementia. They are most effective in mild to moderate stages.
-
Side Effects: ChEIs can cause side effects like nausea, vomiting, diarrhea, and muscle cramps.
Side Effects:
While cholinesterase inhibitors can improve cognitive function and alleviate symptoms in dementia patients, they are also associated with a broad range of potential side effects. These side effects result from the increased activity of acetylcholine throughout the body and can affect various systems, including the cardiovascular, gastrointestinal, musculoskeletal, genitourinary, respiratory, dermatological, psychiatric, and ocular systems. It's important for healthcare providers
to monitor patients closely and manage any adverse effects that arise during treatment.
Biochemical/physiological mechanism of Glutamate Regulator
Glutamate regulators, also known as NMDA receptor antagonists, are a class of drugs used to treat dementia, particularly moderate to severe Alzheimer's disease. They work by modulating the glutamatergic system, which is involved in learning, memory, and other cognitive functions. However, excessive glutamate activity can be neurotoxic and contribute to neuronal damage. Here's how glutamate regulators work:
Biochemical Mechanism:
-
Glutamate and NMDA Receptors: Glutamate is the most abundant excitatory neurotransmitter in the brain. It binds to various receptors, including NMDA (N-methyl-D-aspartate) receptors. These receptors play a critical role in synaptic plasticity, which is essential for learning and memory.
-
Excitotoxicity: In dementia, there is often an imbalance in glutamate levels, leading to excessive activation of NMDA receptors. This overstimulation can trigger a cascade of events known as excitotoxicity, which involves calcium influx into neurons, leading to neuronal damage and death.
-
NMDA Receptor Antagonism: Glutamate regulators, like memantine, act as NMDA receptor antagonists. They bind to the NMDA receptors and block the excessive influx of calcium into neurons, thus preventing excitotoxicity.
Physiological Mechanism:
-
Neuroprotection: By reducing excitotoxicity, glutamate regulators help to protect neurons from damage and death. This neuroprotective effect can slow down the progression of dementia and preserve cognitive function.
-
Synaptic Function: Glutamate regulators can also modulate synaptic function, improving the efficiency of communication between neurons. This can enhance cognitive processes like memory and learning.
-
Anti-inflammatory Effects: Some glutamate regulators may have anti-inflammatory properties, which can further protect neurons from damage and promote their survival.
Limitations:
-
Symptomatic Treatment: Glutamate regulators do not cure dementia or reverse the underlying neurodegenerative process. They primarily offer symptomatic relief and may slow down cognitive decline.
-
Limited Efficacy: The effectiveness of glutamate regulators varies among individuals and may be limited in advanced stages of dementia.
-
Side Effects: Glutamate regulators can cause side effects like dizziness, headache, confusion, and constipation.
Side Effects:
Glutamate regulators, while helpful in managing dementia symptoms, may cause side effects in some individuals. These side effects can include dizziness, headaches, confusion, and constipation. Some people may also experience agitation or hallucinations.
Biochemical/physiological mechanism of Amylod Beta Removal
Amyloid removal medications, particularly those targeting amyloid-beta (Aβ) plaques, aim to reduce the amyloid burden in the brains of patients with Alzheimer's disease. These medications can work through various mechanisms, including immunotherapy (both passive and active) and small molecule inhibitors.
Inhibition of Aβ Production:
These drugs aim to reduce the generation of Aβ peptides by interfering with the enzymes involved in its production. One prominent example is the class of drugs called BACE inhibitors (beta-secretase inhibitors). Beta-secretase is an enzyme that cleaves the amyloid precursor protein (APP), leading to the formation of Aβ. By inhibiting this enzyme, BACE inhibitors can lower the overall production of Aβ.
Enhancement of Aβ Clearance:
This approach focuses on increasing the removal of existing Aβ plaques from the brain. Several mechanisms are being explored:
-
Immunotherapy: Monoclonal antibodies, such as aducanumab (Aduhelm), target and bind to Aβ, promoting its clearance by microglia (immune cells in the brain) through phagocytosis (engulfing and destroying the plaques).
-
Activation of Aβ degrading enzymes: Some drugs are designed to activate enzymes like neprilysin, which naturally break down Aβ. By enhancing their activity, these drugs can accelerate the clearance of Aβ.
-
Modulation of Aβ transport: Aβ is transported across the blood-brain barrier. Certain drugs aim to enhance this transport, leading to increased removal of Aβ from the brain.
Small Molecule Inhibitors:
Small molecule inhibitors are a class of therapeutic agents designed to interfere with specific molecular targets within cells. These compounds are typically low in molecular weight, allowing them to easily enter cells and interact with proteins, enzymes, or other molecules to modulate their activity.
Enhancing Aβ Clearance:
Enhancing amyloid-beta (Aβ) clearance involves strategies aimed at increasing the removal of Aβ peptides from the brain, thereby reducing amyloid plaque burden and potentially slowing the progression of Alzheimer's disease
Reducing Aβ Aggregation:
Reducing amyloid-beta (Aβ) aggregation focuses on preventing Aβ peptides from clustering into toxic oligomers and plaques, which are key pathological features of Alzheimer's disease. This can be achieved using small molecules, peptides, or other agents designed to inhibit the initial formation of Aβ aggregates or disrupt existing aggregates, thereby potentially mitigating neurotoxicity and slowing disease progression.
Physiological Impact:
Amyloid-beta (Aβ) clearance strategies are designed to reduce the accumulation of amyloid plaques in the brain, a hallmark of Alzheimer's disease. By targeting the production, aggregation, or removal of Aβ, these therapies aim to alleviate the detrimental effects of amyloid on brain function and neuronal health.
The physiological impact of effective Aβ clearance can be significant, leading to various benefits:
-
Plaque Reduction: The primary goal of Aβ clearance therapies is to decrease the number and size of amyloid plaques. This reduction can occur through inhibiting Aβ production, preventing its aggregation, or enhancing its removal from the brain. By lowering the amyloid burden, these strategies aim to create a healthier brain environment.
-
Cognitive Improvement: Reducing Aβ accumulation has been linked to improvements in cognitive function in Alzheimer's patients. By mitigating the toxic effects of Aβ on neurons and synapses, these therapies may enhance memory, attention, and other cognitive processes, potentially slowing the progression of the disease.
-
Neuroprotection: Lowering Aβ levels can have neuroprotective effects by reducing neuroinflammation, synaptic dysfunction, and neuronal loss. Aβ is known to trigger inflammatory responses, disrupt communication between neurons, and contribute to cell death. By mitigating these processes, Aβ clearance strategies can help protect the brain and preserve its function.
While the exact mechanisms and long-term effects of Aβ clearance are still under investigation, the potential physiological impact of these therapies is promising. Ongoing research and clinical trials continue to explore various strategies for Aβ clearance, aiming to develop effective treatments for Alzheimer's disease and improve the lives of those affected.
Reference
- Dementia - Definition, Symptoms, Causes
- Alzheimer
- Dementia Brain Scan
- Medications
- Amyloid Plaque
YouTube
|
|